Experimental Research:
Experimental laboratory investigations have focused on rupture propagation, high speed friction, and characterization of elastic properties of rocks. Simulations of fast, subsonic shear ruptures have been (slower than the speed of shear waves) in photoelastic material in the Rosakis lab at Caltech. These experimental ruptures are analogues for earthquake ruptures in crystalline rocks. The dynamic stress field evolution during the experiments are recorded using photoelasticity and high speed photography, so the stress perturbations caused by the rupture can be observed as they change with time on the scale of microseconds.
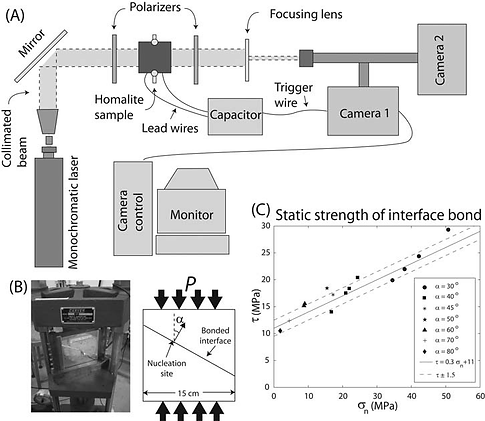
A: Photoelastic apparatus including laser light source, polarizing fi lters, and highspeed cameras. B: Hydraulic press and sample confi guration showing relative orientation of vertical load (P) and inclination of interface (B). Explosion induces a bilateral right-lateral shear rupture upon explosion. C: To constrain the strength envelope of the interface we conducted static strength tests on 14 Homalite samples with glued interfaces at angles ranging from 30° to 80° using an Instron electromechanical testing machine. See Griffith et al, Geology, 2009 for more details.
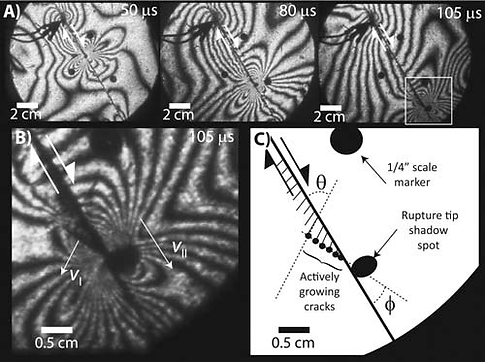
Isochromatic fringe patterns around a shear rupture nucleating at an exploding wire and propagating along bonded interface. Note that fringes surrounding rupture tip expand as rupture grows, indicating an increasing stress concentration with distance. See Griffith et al, Geology, 2009 for more details.
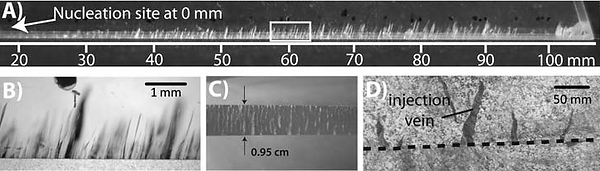
Tensile microcracks in Homalite 100 specimen compared to pseudotachylyte injection veins in the field. See Griffith et al, Geology, 2009 and Ngo et al., JGR, 2012 for more details.
These experiments have been used in conjunction with solutions of analytical boundary value problems to gain insight into the mechanics of dynamic rupture propagation and to constrain the contribution of the dynamic rupture tip to off-fault damage (i.e. fractures). A major goal of this research is to develop criteria for interpreting dynamic parameters (e.g. rupture velocity, stress state) for ancient earthquake ruptures on exposed faults (Griffith et al., Geology, 2009), and to understand the energy budget associated with dynamic damage production.
This work has yielded a mathematical description for the formation of the off-fault tensile cracks that formed during the dynamic experimental shear rupture (Griffith et al.,2009; Ngo et al., 2012). In the study, a fracture mechanics-based analytical description for steady-state sub-Rayleigh shear rupture propagation was developed utilizing a velocity-weakening cohesive zone model.
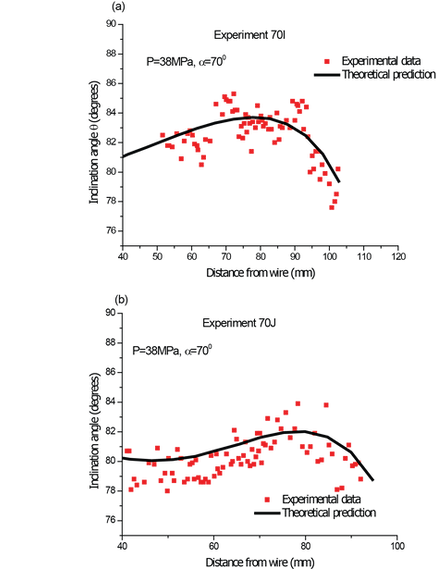
Inclination angle of microcracks formed during two dynamic rupture experiments compared with theoretically-predicted orientation from analytic model developed by Ngo et al., JGR, 2012. Note that variation in inclination angle is tied to variations in shear rupture velocity during experiments. See Ngo et al., JGR, 2012 for more information.
For various assumed velocity-weakening friction laws, the dynamic shear rupture model predicts the occurrence and orientation of the tensile cracks which form in the surrounding material due to transient stress perturbations associated with the passage of the rupture tip. An important feature of the velocity weakening description for dynamic friction used in the analysis is that the velocity weakening parameter, (which describes the linear dependence of crack-tip shear traction and sliding rate), can be back-calculated from the orientation of the observed off-fault micro-cracks. Currently, little-to-no experimental data exists to estimate this velocity weakening parameter directly for the experimental material (Homalite-100) or for crustal rocks, particularly at relevant slip speeds (1-10 m/s) and normal stress ( > 50 MPa).
Understanding the frictional behavior of the rock-on-rock interface in this slip velocity – normal stress parameter space is critical to the physics of the earthquake rupture front. There is broad agreement amongst researchers in the geophysics community that similar rocks may undergo very different weakening processes in different normal stress and/or slip velocity regimes. Consequently, inference of weakening behavior of fault rocks in situ from laboratory experiments at interfacial conditions of relevance to earthquake physics cannot be done simply by scaling exercises, and relatively small changes in normal stress and/or the slip speed can result in changes in the slip weakening distance of an order of magnitude. Motivated by these observations, we (with Vikas Prakash from Case Western Reserve University) are working on a project sponsored by the NSF Geophysics program to advance the current state of our understanding regarding the frictional constitutive behavior of earthquake faults using two principal approaches: (1) implementing a new dynamic shear friction testing apparatus by synergistically combining the split-Hopkinson pressure bar and the double-direct shear friction apparatus to the study of dynamic friction in both intact and granular geo-materials; and (2) developing a methodology for testing the efficacy of parameters extracted from dynamic friction experiments in dynamic rupture models.